The presented contribution depicts the influence of natural and anthropogenic disturbances in the regeneration ecology of Andean, African and Pacific mountain forest systems by comparing examples from Southern Ecuador, Northern Peru, the Hawaiian Islands, Cameroon, and Eastern Africa.
All systems show a very comparable forest zonation, with mosaic-climax formations dominating the lower montane zone, and monotypic forests taking over at higher altitudes. Neotropical mountain forests harbor much higher species diversity, mainly due to the extreme radiation of trees and epiphytes, particularly Orchids and Bromeliads. In Africa, herbs are much more abundant due to the availability of more large gaps created by large mammals, and in the Central Pacific, island bio-geographic processes have the highest influence on forest species diversity.
Similar regeneration processes can be observed in all systems, although the role of natural landslides is much more important in the Andes and the Pacific region, whereas fire and big-game influence are the dominant motors for regeneration in Africa. The impact of antropogenic influences, e.g. slash and burn, timber extraction, exploitation of forest products, introduction of exotic species etc. on the regeneration and succession patterns in Tropical Mountain Forests are discussed, and possibilities for future management depicted.
Entre los puntos calientes de biodiversidad mundial, los bosques montanos tropicales tienen un papel especialmente importante. Estos sistemas contienen un numero más alto de especies por area que los bosques humedos, pero estan mucho mas pequeños, seriamente fragmentados, y tienen una funcion muy importante como captaciones de agua y bareras contra la erosion.
Este articulo ilumina la influencia de perturbaciones naturals y antropicas por la ecologia de regeneracion de bosques montanos Andinos, Africanos y Pacificos, comparando ejempos desde Ecuador, Peru, Hawaii, Cameroon y el Este de África.
Todos estos sistemas muestran una zonacion de bosque muy comparable, con formaciones del tipo climax-mosaica en la zona baja y bosques monotipicos como unidades mas importantes en las zonas altas. La diversidad de especies en los bosques Neotropicales es mucho mas alto, especialmente por la radiacion de arboles y epifitas, en particular Orquideas y Bromelias. En África hierbas son mucho mas abundante por la disponibilidad de claros largos, causados por la actividad de la megafauna y en el Pacifico Central procesos de biogeografia insular tienen la influencia mas alta por la diversidad de especies.
Introduction 
Tropical
mountain forests, found mainly at altitudes between 1200 and 3500 m
(LaBastille & Pool 1978, Stadtmüller 1987, FAO 1992) are
amongst the species richest ecosystems worldwide. Particularly the
Eastern Andean Region represents one of the hottest
"biodiversity-hotspots (Myers et. al. 2000). In striking
contrast to tropical lowland rainforests, these tropical montane
forests have received only marginal attendance in science and society
until recently, despite their ecological and economical importance as
water catchments and erosion barriers. The neotropical montane region
is considered one of the twelve major crop-gene centers of the world
(Churchill et al. 1995). At the same time, mountain forests are
especially sensitive ecosystems due to their steep relief, which
allows extreme erosion under a high rainfall regime. Due to increased
population pressure and resource use (firewood, mineral resources,
pastures, agriculture), montane forests are more and more rapidly
dwindling. FAO (1993) estimates that tropical mountain forests
comprise about 11 % of the worlds tropical forest resources, and
suffer an annual deforestation of about 1.1 %. Mountain forests
generally occur on more humid mountains, and most frequently in Latin
America and Southeast Asia, where the most widespread mountain areas
occur, with outposts in tropical Africa (Stadtmüller 1987).
Most studies carried out in tropical ecosystems have focused on the lowland rainforests, and most research stations are located there (Leigh 1999). Even there, very little is known about the regeneration processes in the ecosystem (Finegan 1996), and fairly nothing about its functioning. In tropical Mountain Ecosystems, studies concentrated mainly on the alpine zone, whereas the often-inaccessible forest belt with its extreme species richness has hardly been studied. The African and Andean zone make no exception. Although particularly Andean forests host an extreme , - and - diversity (Barthlott et al. 1996; Ibisch 1996), often comparable or higher than species counts for Amazonian areas (Balslev. et al. 1998), no comprehensive studies of a tropical mountain forest ecosystem have been undertaken. Even broad-focused projects like "Ecoandes" in Colombia (Hammen et. al. 1983, 1984, 1989a, 1995) included the forest regions only marginally. First attempts to bring the frugal knowledge existing together were made only recently (Hamilton et al. 1994, Churchill et al. 1995). The deficit of scientific knowledge particularly includes all aspects of regeneration of montane forests, as well as succession processes after any kind of natural or man-made impact. The habitat requirements and the potential for rejuvenation of important tree species are almost unknown.
The present work focuses on a comparison of various Andean, African and Pacific montane forest ecosystems, as examples for tropical mountain forests in general.
Materials and Methods 

Study Areas
The
border region of Ecuador and Peru is one of the most biologically
diverse areas worldwide, and thus a "biodiversity-hotspot"
par excellence. Low passes in the Andean chain allow an easy exchange
between the floras and faunas of the Amazon Basin and the pacific
lowlands. Additionally, the region shows a very fast transition
between the humid mountain forests of the northern Andes and the dry,
deciduous forests of the northern Peruvian lowlands. Until the recent
past, the Podocarpus National Park and the study area have been
almost unknown scientifically. The most recent new approach for the
classification of the vegetation of Ecuador (Sierra 1999) lists all
montane forests between 1800 - 3000 m altitude as "bosque
de neblina montano", without further distinction. In the
Southern part of Ecuador and the North of Peru, a number of lower
ridges with deep and dry valleys are found. The area is the lowest
part of the Andes near the equator. Particularly the peak regions are
covered by dense clouds or mist most time of the year. The main
rainfall occurs in February-March and particularly June-September,
both periods followed by a dry season, which can lead to a negative
water balance especially during months from October-January.The natural forests of Africa have been subjected to heavy tree felling for decades. Moreover, due to selective logging, they also have undergone significant changes of their species composition. Although private tree planting of exotic species has reduced the fuelwood deficit considerably, the destruction of the natural forests has increased drastically in the last decades. If current rates of forest clearing continue, no natural forest will remain by the year 2040 (Barnes 1990).
The climate of Africa shows the characteristic pattern of inner tropical regions with large diurnal temperature oscillations, known as "Tageszeitenklima" (Troll 1959), but a small amplitude of the monthly means in the course of the year. The "long rains" last from March to June, while the "short rains" occur from October to November. Due to the equatorial position, the daylight period is nearly constant all year round, which is an important factor for vegetation growth.
The results of the phytosociological fieldwork and ecological observations conducted in Kenya and Ethiopia from 1992-2004 have been presented in a wide array of publications (Bussmann 1997, 1999, 2001a,b, 2002; Bussmann & Beck 1995a,b,c; Bussmann & Lange 2000; Bytebier & Bussmann 2000; Lange et al. 1997).
Mount Cameroon is located on the coastal belt of the Gulf of Guinea in the South-West of Cameroon, rising steeply from the coast to a height of 4095 m. The summit lies only 20 km inland. It is part of a chain of volcanic mountains, covered by a tropical montane forest. This chain stretches from 100km South-West of Mount Cameroon, from the Islands of Anobon to the highlands of the Adamawa in North-Western Cameroon to Obudu in Nigeria.
Remaining natural-like forests in the Hawaiian Islands and French Polynesia were visited for comparison to the other Mountain Forest sites studied in detail.
Methodology
For
the phytosociological analysis of the African mountain forests in
Kenya, Ethiopia and Cameroon from 1992-2004, more than 1250 relevés
were established and analyzed. In Ecuador and Peru, starting 1995,
after a detailed floristic inventory based on random samples, 400
plant-sociological relevés were established in
physiognomically homogenous forest areas, and 150 plots on natural,
as well as 200 plots on antropogenically induced landslides were
established. All Plots were selected and sampled according to the
methods of Braun-Blanquet (1964) and Mueller-Dombois & Ellenberg
(1974), as slightly modified by Hammen et al. (1989b). All relevés
were sampled at least twice and in different seasons, for which
reason the species inventory should be more or less complete in most
cases.The collection of environmental data on landslides included soil texture of the upper mineral layer, and the soil pH. The depth of the humus layer was measured as an important indicator of successional age and ongoing erosion. The inclination and position on the slide was recorded as well as the altitude above sea level, the direction aspect and the geographical position of the landslide. Space-for-time substitution (Pickett 1989) was employed to describe successional processes of initial stages. Knowledge about the history of the slides can be gained by studying the aerial pictures of the regions. However, the time since the last major sliding event for the plots could not be assessed accurately because most of the landslide material is not displaced by one big event but by several consecutive "ongoing" slides Stoyan (2000). Further on, many of the slides were invisible at the aerial pictures due to their small size and the steep relief.
During the extensive phytosociological fieldwork special attention was given to regeneration patterns and succession processes in the forests, these observations leading to the regeneration cycles presented here.
Results and Discussion 

Forest vegetation units in Africa, Latin America and the
Pacific
Extremely steep slopes and deeply incised riverine valleys, providing a mosaic of different microclimates, as well as frequent natural landslides, lead to a very variable mosaic of vegetation units in the Andean region. Based on the dominance-oriented floristic data the montane forests of the area have been grouped into three main formations, each covering a specific altitudinal range.
The prevalent forest formation in South Ecuador and North Peru at altitudes from 1000 - 2100 m, with an extremely diverse, 2-storeyed tree stratum, reaching 25 m, with emergent trees up to 35 m tall in riverine valleys and mean tree of 100%, is the "Lower Montane broad-leaved forest" (Ocotea - Nectandra forest) (Bussmann 2001c, 2003), extending up to 2300 m at the bottom of wind-protected riverine valleys. Species belonging to families found mainly at lower altitudes (e.g. Cyclanthaceae, Lauraceae, Hymenophyllaceae) are very common in this formation, whereas representatives of the flora of higher regions are very rare. Undisturbed tracts of the Ocotea-Nectandra forests can be mainly encountered on very steep slopes with an inclination of 30-50° or more, as well as in almost inaccessible valleys. In areas easier to reach, this formation has been almost entirely destroyed by human activities, and has been replaced by secondary forest. The epiphyte- and ground-flora contains especially ferns (particularly Lomariopsidaceae) and Orchidaceae. In contrast to higher altitudes, Bromeliaceae are less frequent. The natural regeneration of gaps in these forests leads to a typical mosaic-climax. Where the climax forest has been destroyed by natural fires the tree stratum is dominated by large palms (Arecaceae, Dictyocaryum lamarckianum). Characteristically, the herb stratum is dominated by different species of bamboo (Chusquea spp.), as well as large Gleicheniaceae forming almost impenetrable thickets. The regeneration of the climax species seems to be inhibited in these areas. In areas with formerly strong human influence (slash-and-burn, clearcutting), a completely different, monotypic secondary forest develops. These forests have one tree stratum, completely dominated by the 10-12 m tall stems of Axinea quitensis (Melastomataceae). Species diversity is much lower. Almost no epiphyte species are encountered, and the ground flora is extremely impoverished.
At altitudes above 2100 m, up to about 2750 m, the "Upper Montane Forest" (Purdiaea nutans - Myrica pubescens - Myrsine andina forest) (Bussmann 2001c, 2003), a monotypic formation, with only one tree stratum, with stems between 5-10 m, sometimes up to 15 m tall, replaces the Ocotea-Nectandra forest. Lowland species are completely disappearing. The canopy is completely dominated by the twisted stems of Purdiaea nutans (Cyrillaceae), which has its main distribution in northern Peru. A very diverse stratum of small treelets and shrubs occurs, and the undergrowth is often completely dominated by large Bromeliads. Above 2450 m, particularly on wind-exposed ridges, a transition to the "subalpine elfin forest" can be observed. Frequent landslides foster the dynamics in the upper montane forest, and are inevitable for the maintenance of its high floristic diversity. In overaged forests, species diversity declines drastically. On natural clearings, in flat ridgetop areas, which are most probably the effect of fires, the tree stratum almost disappears, making way for a dense layer of grasses, suppressing almost all other species in the ground layer. In extremely dry years, fires occur also on steeper, wetter slopes.
The uppermost forest belt is formed by the "Subalpine-Elfin Forest" (Bussmann 2001c, 2003), which closely resembles the Bolivian "Yalca". This formation - more like an impenetrable bushland than a forest, is closely dovetailed with the adjacent Páramo region. The "timberline" in the area is mainly induced by strong winds. Wind-protected areas are densely covered with Yalca vegetation, whereas more open, wind-exposed regions at the same altitude are covered with grassy Páramo vegetation. A real timberline thus does not exist in the study area. The only 1-2 m wide crowns of the dominant species - particularly Cunoniaceae, Clusiaceae, Clethraceae and many small Melastomataceae, form a very dense canopy, allowing only little light to reach the ground. The stems of these "trees" protrude thus from a literally meter-deep carpet of mosses, whereas in contrast to the rich woody flora almost no herbal species are found in the ground layer.
In East Africa, the montane forest vegetation has been studied intensively in the last decade (Bussmann 1997, 1999, 2001a,b2002; Bussmann & Beck 1995a,b,c; Bussmann & Lange 2000; Bytebier & Bussmann 2000; Lange et al. 1997). The Evergreen Submontane Forest with its two storied tree layer are found mainly on the very wet south-eastern and southern slopes of Mount Kenya on altitudes between 1550-2550 m, growing on humic Niti- and Acrisols and receiving an annual rainfall of 1500-2500 mm.
In contrast to Latin America, a wider array of montane forest communities can be found. The Evergreen and Evergreen Xeromorphic Montane Forests growing on humic Acrisols (Speck 1986) with rainfall between 700 and 1500 mm, represent the typical vegetation of the altitudinal range between 2500 and 2950 m. In these forests, the pencil Cedar (Juniperus procera) grows about 50 m tall under favorable conditions. Other important tree species, mainly of the lower canopy, are the African Olive (Olea europaea ssp. africana) and Podocarpus latifolius. Evergreen Montane Bamboo Forests, dominated by the African Bamboo, Sinarundinaria alpina, are found on various East African mountains. Bamboo forests are restricted to a relatively narrow ecological range, mainly depending on a soil temperature of 10-15 °C and the presence of very deep volcanic soils, namely humic Andosols. The cyclic changes of the bamboo vegetation lead to regular changes of the floristic composition. One single growth cycle of Sinarundinaria alpina is supposed to take 15 to about 40 years (Wimbush 1945; Agnew 1985, and own observations give indications for even longer periods).
Finally, the timberline is formed by the Evergreen Subalpine Forests, extending from 2900 to 3300 m a.s.l., where low clouds and mist are frequent. The dominant tree is the "Kosso"-tree, Hagenia abyssinica (Bruce) J.G. Gmel., of the Rose family.
The vegetation of Mt Cameroon is continuous and largely unbroken from sea level to the summit. Zonation falls in lowland evergreen forest (0 - 800 m), sub-montane forest (800 - 1700 m), montane forest (1600 - 1800 m), montane shrubs (above 1800 m) and sub-alpine grassland (2000 - 3000 m).
Regeneration Ecology of Tropical Montane Forests
Typical Mosaic Climax Regeneration in Tropical Montane
Broadleaved Forests
Only few natural remnants of lower montane forests remain after centuries of logging. Under natural conditions these forests show a typical mosaic-regeneration cycle, where the gaps resulting from natural treefall or larger natural clearings are randomly closed by the species arriving first. In most cases however, a typical set of fast growing pioneer species acts as shade trees, under which the typical, shade tolerant climax species develop [[(Figure 1)]]. In Southern Ecuadorian Lower Montane Forests, gaps are rapidly colonized by Cecropiaceae (particularly Cecropia montana with its shiny silvery leaves), Piptocoma discolor (Asteraceae), Isertia laevis (Rubiaceae) and Heliocarpus americanus (Tiliaceae). Rapidly the canopy is being closed again, and climax species are developing.
Regeneration processes in Africa are less simple. The East African Lower Montane Forests are characterized by huge Camphor trees (Ocotea usambarensis). This most characteristic tree of this vegetation type, is widespread on East African mountains (White 1978, 1983; Friis 1992), where it inhabits an altitudinal range between 1300-2600 m. The species is heavily logged for its valuable hardwood, but natural regeneration is problematic. Willan (1965) described the difficulties to regenerate Ocotea on the southern slopes of Kilimanjaro and in the West Usambara Mountains. According to his reports, Macaranga is dominant in gaps where Ocotea is absent, i.e. has died naturally or has been logged out. Saplings of Ocotea are successfully competed by Macaranga, a fastly growing pioneer species, so Willan proposed a removal of all Macaranga trees before replanting Ocotea.
Surveys on Mt. Kenya (Bussmann 2001b) revealed, that the remaining stands are almost exclusively composed of very old Ocotea trees of approximately 300-600 years of age (stem diameter 1.2-2.5 m, estimated according to Bussmann 1999). Very few young trees (less than 100 years old) and seedlings were encountered. In that region, the main flowering of the dioecious Ocotea usambarensis occurs between early March and late April, at the beginning of the long rains. A second flowering can be observed after the start of the short rains in November. In most years, however, only few trees flower, whereas every five to seven years mass-flowering occurs. Data for O. kenyensis in Ethiopia suggest, that the number of seeds and seedlings is relatively large in such years, and seedling density might reach up to >700/ ha (Getachew et al. 2002). A large fraction of seeds are attacked by gall-insects and birds, while seedlings suffer heavily from herbivory. Germination rates are high in the shady conditions indicating the late successional character of the species. The seeds are only viable for a few days, even under the most favorable conditions like high humidity and radiation (Katende et al. 1995; Bussmann & Lange 2000). Under natural conditions, few seeds manage to establish (Bussmann & Beck 1995a,b). This explains the often found uniform age structure, although continuous regeneration was also described (Getachew et al. 2002). Ocotea effectively regenerates with suckers growing from stem bases and old roots. This contrasts with the other characteristic tree species of these forests, that mostly depend on sexual regeneration. Even-aged stands of old Ocotea usambarensis trees die over an extended period of time, creating a mosaic of gaps in which the Euphorbiaceae Macaranga kilimandscharica start growth as a secondary species. This leads to patches of monotonous vegetation, but only on small scale, and does not change the structure of the forest to a significant extent. Sexual regeneration of Ocotea from seedlings depends on the coincidence of mast years with suitable conditions for seedling establishment, and on the level of herbivory, but usually numerous suckers from the roots of a fallen tree, not longer suppressed by the mother tree, start growing. Macaranga kilimandscharica hereby plays an important role as a shade tree, as Ocotea seeds and saplings do not tolerate full sun. After the break down of the relatively short-lived Macaranga trees, the meanwhile well established young Ocotea trees close the gaps, and effectively prevent further germination or establishment of M. kilimandscharica by shading. Thus, Ocotea stands of a more or less uniform age structure result.
On many mountains, Ocotea usambarensis is the most heavily exploited species (Lambrechts et al. 2002). The typical practice of clear-cutting by means of large scale uprooting of the trees hampers vegetative regeneration of Camphor. The big gaps are fastly closed by Macaranga kilimandscharica and at higher altitudes by Neoboutonia macrocalyx. As Camphor does not regenerate in these man-made "gaps" after breakdown of the secondary trees due to the lack of diaspores, these forests regenerate in a cycle of their own. The possibilities for a regeneration to primary forest are unknown, and presumably require very long time spans. In any case, a complete logging stop around the gaps seems crucial to allow colonization.
As pointed out, seeds, seedlings and suckers suffer from herbivory. Unfortunately, the influence of megaherbivores on vegetation has mainly been studied in savannah ecosystems (Buechner & Dawkins 1961, Kortland 1984), and only few data exist on forest habitats (Holloway 1965, Schmitt 1991, Schmitt & Beck 1992, Plumptre 1993; Reed 1997). However, logging certainly is more important that herbivory.
The lower montane forest in the Hawaiian Islands have been almost entirely destroyed. Originally, Metrosideros polymorpha (Myrtaceae) and Acacia koa (Mimosaceae) formed a monotypic canopy. This monotypic climax, a very special case for a lower montane forests shows a regeneration cycle very comparable to higher altitude formations, and is thus treated in the following section.
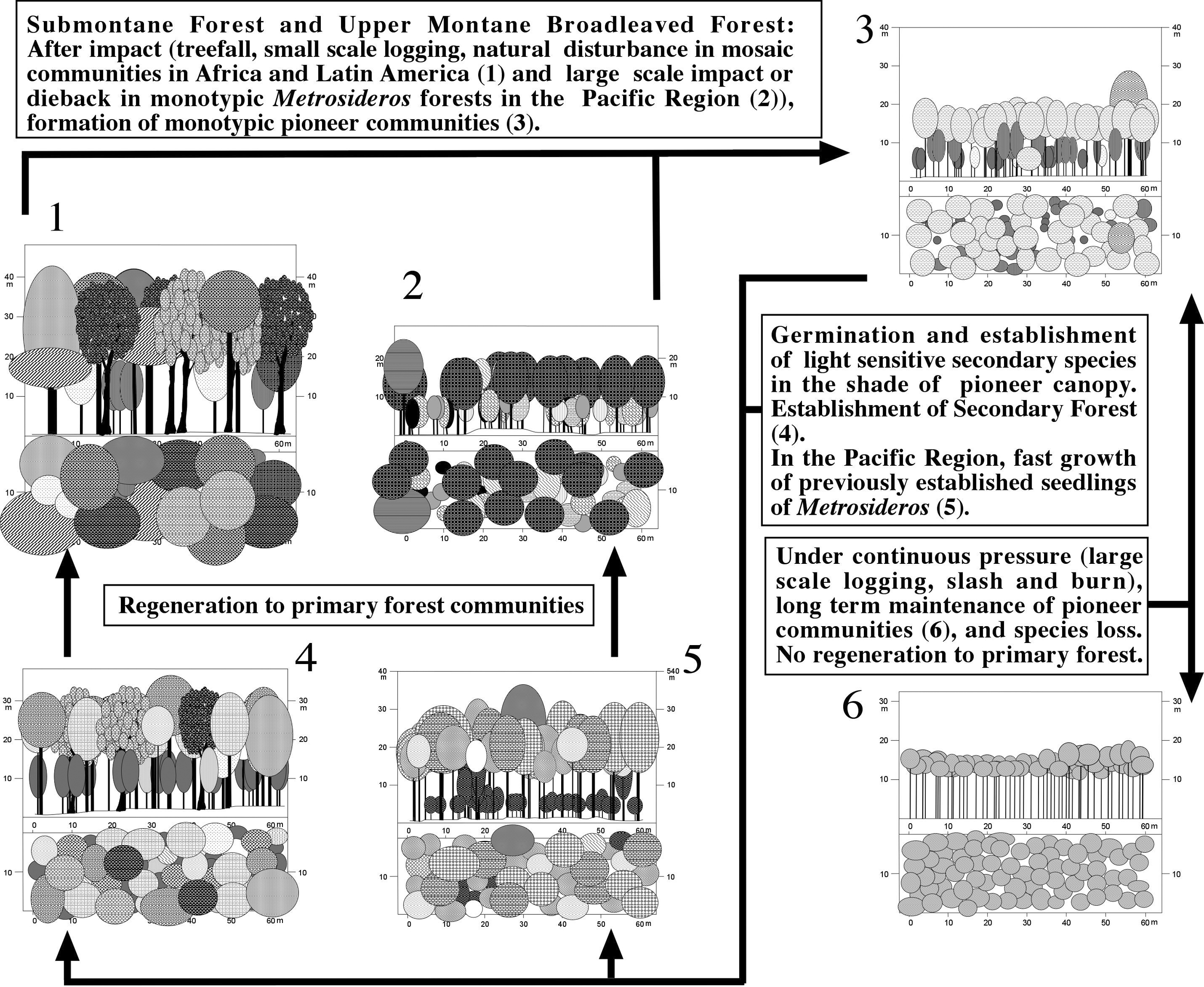
Figure 1:
Regeneration Processes in Tropical Lower Montane Forests.
Figura 1: Procesos de regeneración en el Bosque Montano Bajo.
Figura 1: Procesos de regeneración en el Bosque Montano Bajo.
Regeneration of monotypic climax forests
Fire apparently plays an important role in the regeneration cycle of Upper Montane Forests [[(Figure 2)]].
The Montane Xeromorphic and Breadleaved Forests in East Africa are an excellent example for the regeneration strategies of Tropical Clound Forests. Montane broadleaved forests with Cassipourea malosana are locally common at drier afromontane sites. Regeneration generally seems to be continuous, since trees of all age classes were found. All important species lack characteristics of pioneer species, like the capability to build a seed bank, and most of them preferably germinate in the shade (Olea capensis, Popocarpus gracilior; Getachew et al. 2002). Due to the environmental conditions, especially the high rainfall, fires are rare, and, whenever occurring, they are limited in extent. In unburned forest, Juniperus procera occurs only sporadically, as its germination and regeneration is suppressed by heavy shade and dense vegetation covering the forest. Regenerating Cedar was only found in gaps where seedlings compete with those of the broad-leaved species.
Thus, fires play an important role in the regeneration cycle of the Montane Xeromorphic Forest. Juniperus procera, the African Pencil Cedar, typically grows in the drier montane, submontane and subalpine areas of the East African mountains between 1000 and 3000 m (Kerfoot 1961). Temperature as well as rainfall apparently control its distribution (Winiger 1979). In his study of the southern Aberdare Range, Wimbush (1937) suggested that in the absence of fire Juniperus forest is only a serial stage in the succession to a broad-leaved forest. After fire, herbaceous pioneer species and Juniperus procera seedlings invade the gap, leading to a regenerating forest, where broad-leaved species as Olea capensis ssp. hochstetteri, Olea europaea ssp. africana and Olinia rochetiana become also established. Cedar is well adopted to the conditions on forest clearings in the montane belt, since its seeds germinate best in full light and under comparatively low temperatures (15 - 20°C, Demel & Granström 1997a; Bussmann & Lange 2000; Eshetu & Leinonen 2002). Seeds were shown to survive for several years in the soil (Demel & Granström 1997b), but showed no apparent dormancy. In contrast, Olea europaea has as strong dormancy, which is broken by scarification of the seed. Germination rates will be almost zero unless the seed coat is is removed. (Legesse 1993). The Olive builds a transitory seed bank, too, and seedlings are abundant under full insolation, although they are heavily browsed (Kebrom & Tesfaye 2000; Getachew et al. 2002). Another important member of this cycle, Nuxia congesta, also develops a transitory seed bank (Demel & Granström 1995).
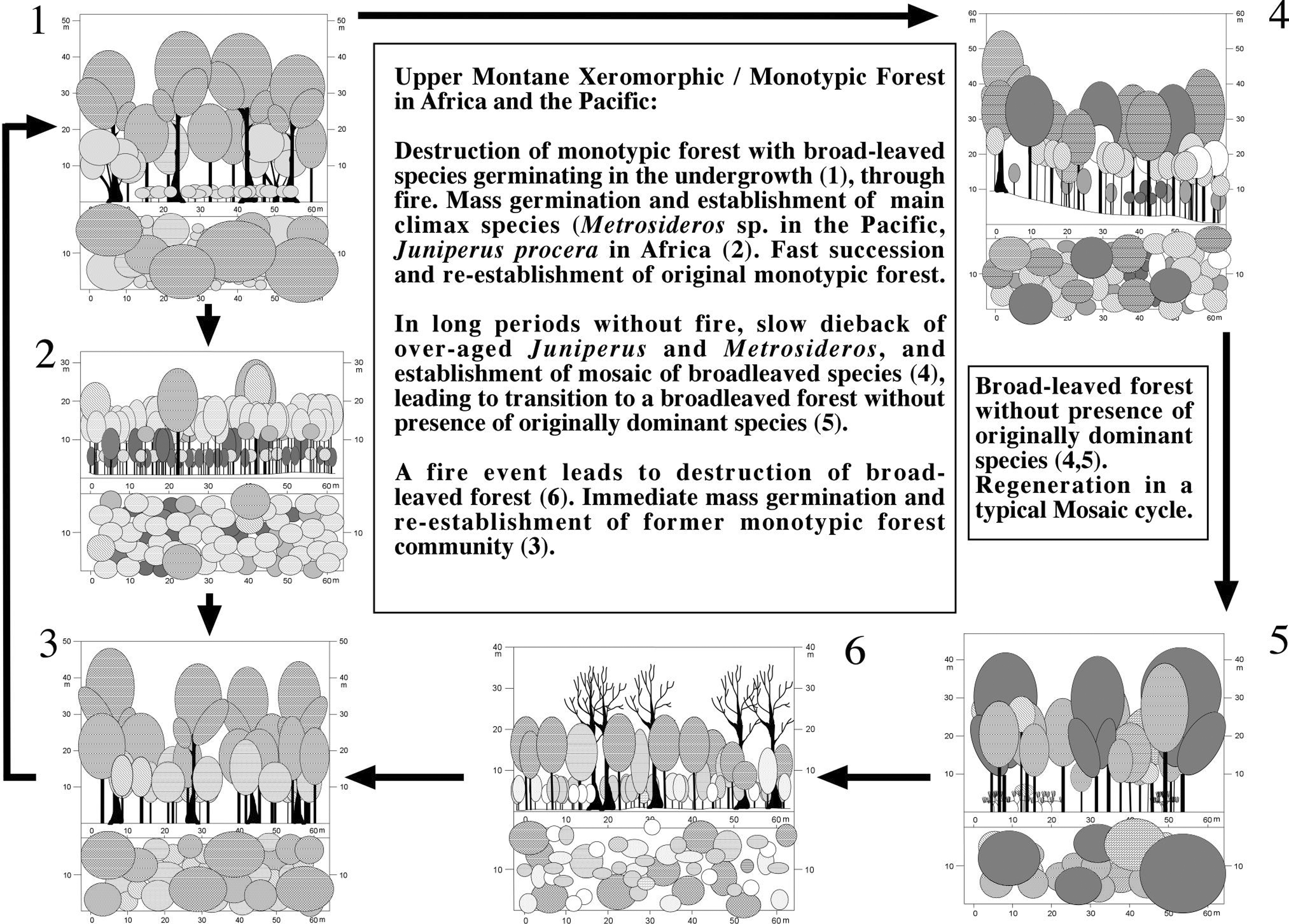
Figure 2:
Regeneration Processes in Tropical Upper Montane Forests.
Figura 2: Procesos de regeneración en el Bosque Montano Alto
Figura 2: Procesos de regeneración en el Bosque Montano Alto
After about 20 years, the Juniperus trees have grown about 9 m high. When a closed canopy is established, the Cedar trees start to outgrow the other species, reaching about 30 m after 80-90 years. If the number of Juniperus trees established after a fire was high, a pure Cedar forest results by suppression of other trees. After about 200 years, the maximum height of about 40-46 m is reached. Although frequently infected by the fungus Fomes juniperinus thinning the crowns, the Juniperus trees may live for another 300-700 years. In case a burnt area is mainly invaded by broad-leaved species, these constitute the canopy and only scattered Juniperus specimens emerge. As Cedar cannot regenerate in closed vegetation, small natural gaps are filled with broad-leaved species. Therefore, pure broad-leaved forests built the climax community after some time. These broad-leaved forests represent a stable climax, if not destroyed by fire (Bussmann & Beck 1995c, Bussmann & Lange 1999, Bussmann 2001a).
In over-aged climax communities of the Ecuadorian Upper Montane Forests, the ground layer is almost entirely dominated by large grasses, particularly Neurolepis elata (Bambusoideae), the canopy gets very depleted and open, with canopy cover as low as 5%. After mass flowering events of Neurolepis, as well as during extremely dry conditions, as occurring during El Niño / La Niña years, a large amount of dry organic matter is being accumulated, and provides ideal conditions for fires. After burning, the resulting open spaces are rapidly invaded by fern species, without a pronounced cryptogamic stage. Within a short time, woody species belonging to the surrounding primary forests start growing. After a few years, the characteristically dominating Bromeliads occur. Due to the very low annual growth of most tree species in this region, a very dense shrub stratum develops and is being maintained for many years. Slowly Purdiaea nutans starts outgrowing all other species, to form the typical monotypic climax. After about 4-500 years this dense tree layer starts dying back, and the dense shrub and bromeliad layer is replaced by the more light-tolerant grass species again.
Cyclic processes in the Bamboo forests
The
African Bamboo, Sinarundinaria alpina, inhabits the highland
and mountain areas of East and Central Africa at altitudes between
1800 and 3400 m (Hedberg 1951; Dale & Greenway 1961). Bamboo
forests prefer deep volcanic soils, especially Andosols (Speck 1986
and own observations). According to Wimbush (1945), Bamboo shoots can
reach a height of up to 18 m and a stem diameter of up to 10 cm. New
shoots are sprouting from old rhizomes every two to three years. The
main growing period starts some weeks after the long rains and lasts
for about one to two months. New shoots are to be encountered after
each long rainy season. However, maximum rates of biomass production
with most of the 4500-7000 culms being replaced per hectare require
longer periods of favorable climatic conditions. When old culms die
and fall over, young sprouts from the dense network of old rhizomes
will replace them, thus giving rise to a homogenous Bamboo forests.
In undisturbed areas, where the lifespan of a culm is estimated at
about fourteen years, the numbers of dying culms roughly equal the
new ones. Flowering, after which the culms die, occurs in areas of
about one to several hundred acres in more or less regular intervals
(Wimbush 1945). On Mt. Elgon a flowering cycle of fifteen years was
observed for a given stand, but reported figures differ among
authors. At a given flowering event, only part of the stands in an
area will flower, but occasionally there are mass flowering events
with large-scale synchronization. In most years, only small areas of
flowering or postflowering Bamboo are found, and populations of
different mountain ranges are not synchronized. Thus, mass flowering
events appear to be rare, and this is in clear contrast to many Asian
and South American Bamboo species.
Seeds are said to exist (Greenway in Agnew 1985; Katende et al. 1995), but have not been described in the available literature. Thus, we can not rule out that sexual propagation plays some role, but vegetative regeneration is the principal and overwhelmingly important mechanism. Agnew (1985), based on extensive knowledge of the vegetation of the Aberdare Range and after the analysis of ten 1x1 m quadrates, distinguished a Bamboo cycle with five stages :
1. Pioneer - Regeneration from sections of old rhizomes and rhizome fragments and occasionally from fallen culms, lasting about three to five years, during which dense, 1-3 m tall thickets invade the still present Sambucus africana stands of the previous cycle.
2. Building - During this period, the growing Bamboo exceeds the average plant height in the stand, and the vegetation becomes floristically depleted as the bright light requiring species of the pioneer stage disappear and more shade tolerant species of the following stage, which might be survivors of a previous cycle, get momentum. The pH of the soil starts decreasing.
3. Mature - No further increase in culm height takes place during this stage. The culms show even age classes, and the soil pH is decreasing further.
4. Flowering and 5. Sambucus Stage - Within two or three years after mass-flowering the culms die and fall, so light-demanding species invade, above all Sambucus africana. The soil pH increases again during this stage. This situation offers opportunities for the establishment of several montane tree species such as Podocarpus latifolius, Nuxia congesta and Dombeya goetzii. Later, these often form an upper canopy storey above the mature Bamboo stands.
The Bamboo cycle is an important source of autogenic dynamics, and many species specialize on the distinct site conditions of the various stages. Biomass productivity is generally high, and many, especially large herbivores travel the Bamboo belt regularly. Often, their large trails are the only opportunity to pass through the otherwise almost impenetrable forest. The high production of durable and relatively easy to handle "wood", made Bamboo one the preferred building materials for the local population. Moreover, in some places shoots are collected and form an important source of carbohydrates at the end of the rainy season, when crops are planted but not yet ripe. Not surprisingly, many Bamboo stands suffer from heavy exploitation (Scott 1994; Banana & Tweheyo 2001).
On Mt. Kenya, the neighboring Aberdare Range, and on Mt. Elgon, Bamboo stands grow mainly on the wetter western, southern and southeastern slopes and are largely absent in the Northern areas. Sinarundinaria alpina appears to require annual precipitation totals of about 800-1000 mm per year, although arid periods are tolerated. On Mt. Kilimanjaro Bamboo is strangely absent (Grimshaw 1999). Of special interest is the occurrence of large areas of Bamboo forests in Ethiopia. They occur at several sites, albeit with limited extent (Central Ethiopia and Harenna Forest: Tamrat 1993; Bussmann 1997, Illubabor: Friis, pers. comm.). The large Bamboo populations of South and Central Kenya (Mt. Kenya, Aberdares, Mau, about 1000 km South of Harenna) are linked to Harenna only by a small population occurring on top of Mt. Nyiro at the southern end of Lake Turkana, which is also at least 500 km away from the Bale mountains (Bytebier & Bussmann 2000). Because the importance of sexual propagation is doubtful for this species, means of dispersal over such long distances are even less clear than for other vascular plants. Therefore the large gaps between the main population and these outposts pose some interesting questions regarding the ecology and former distribution of Sinarundinaria, as influenced by the palaeoclimate of the region. This requires further fieldwork, especially since some of the much more easily dispersed companions of Bamboo forest are apparently absent from Ethiopia (Sambucus africana; Friis, pers. comm.)
Regeneration in the subalpine forests
The importance of fires for the cyclic regeneration processes in subalpine forests is again high [["Figure 3"]]. Fire cycles can be found in Hagenia abyssinica dominated forests in Africa, Polylepis and other subalpine forests in Latin America, and Metrosideros polymorpha forests in Hawaii. The regeneration processes in Hagenia abyssinica forests have been described more than a decade ago (Schmitt 1991; Bussmann & Beck 1995). This seems surprising, since forests of Hagenia abyssinica are predominately located in the misty regions of the East African mountains, where high precipitation, as well as frequent cloud formations, should prevent plant material from becoming sufficiently dry to burn. Martin (1982), however, stressed that fires tend to be most frequent in areas with a combination of comparatively high rates of primary production, slow decomposition rates, and occasional droughts. Houston (1995) stated, that the alternation of wet and dry seasons produces ideal conditions for fires in semi-humid regions. East Africa is notorious for its unreliable precipitation (Nieuwolt 1978), and the occasional presence of drought conditions triggers large-scale wildlife fires in the East African mountains (Wesche in press). Long-term measurements are rare, but the few records available suggest intervals of droughts of 2-10 years for a given mountain range.
Thus, in sufficiently wet years, the relatively high plant productivity, the large litter production of an adult Hagenia, with its continuously shed leaves (Miehe & Miehe 1994a), and the low decomposition rates in the cold climate of the subalpine forests, result in massive accumulation of fuel. This facilitates extensive fires in drought years, although the frequency a given patch gets burnt certainly is lower then the drought year intervals. The presence of former fires is indicated by charcoal layers, which have been found in soil profiles from Hagenia forests. Field observations revealed, that regeneration stages of Hagenia abyssinica are always growing together with either young seedlings of Juniperus procera and other fire-tolerant plants. Moreover, the even-aged character of many Hagenia forest stands suggest, that the Kosso is not regenerating successively, but all of a sudden after a disturbing event like fire. Germination tests yielded, that Hagenia seeds do not germinate, unless open conditions are available (Lange et al. 1997). Fire is the only natural factor that is able to destroy the undergrowth of larger areas, and to provide an environment with reduced competition, suitable for germination of the seeds. Since germination was promoted by direct light and by temporary heating equivalent to short fires, regeneration of Kosso clearly depends on a fire event.
The climax forest shows adult Kosso trees of uniform size and age, and Juniperus procera as a co--dominant tree species. The canopy of these forests is often closed to nearly 100 %, and the undergrowth consists of a dense herb and grass layer, whereas higher shrubs are rarely found. The conditions of high competition and intensive shading of the ground are not suitable for the germination of Hagenia abyssinica. A fire event disturbs the climax community by destroying herbs and leaf litter in the undergrowth, as well as all roots in the upper soil layers, whereas the adult Kosso trees survive although more or less severely damaged. Miehe & Miehe (1994a) regarded adult Hagenia trees as largely fire-resistant, as their bark is peeling off in large pieces, preventing a fire from reaching the essential vascular strands of the tree. Supporting this assumption, Kosso trees sometimes show heavy signs of former burning, but are still green and even flower. After a fire, the heated ground, now bare of vegetation and only covered with black ash, provides ideal conditions for the germination of the Kosso seeds.
In case the time intervals between two fire events are sufficiently high, the young Hagenia trees are found scattered between a dense pioneer vegetation of about 5 to 6 m height. On Mt. Kenya, Clutia abyssinica (Euphorbiaceae), Dombeya torrida (Sterculiaceae), Helichrysum schimperi (Asteraceae) and Leonotis mollissima (Lamiaceae) are characteristic species in the impenetrable thicket of this successional stage after fire. In contrast, more frequent burning results in a shift to a less high and dense vegetation dominated by Gnidia glauca (Thymeleaceae).The high altitude forests in Latin America and the Pacific follow very much this pattern (Drake et al. 1993; Kessler 2002; Kitayama et al. 1997).
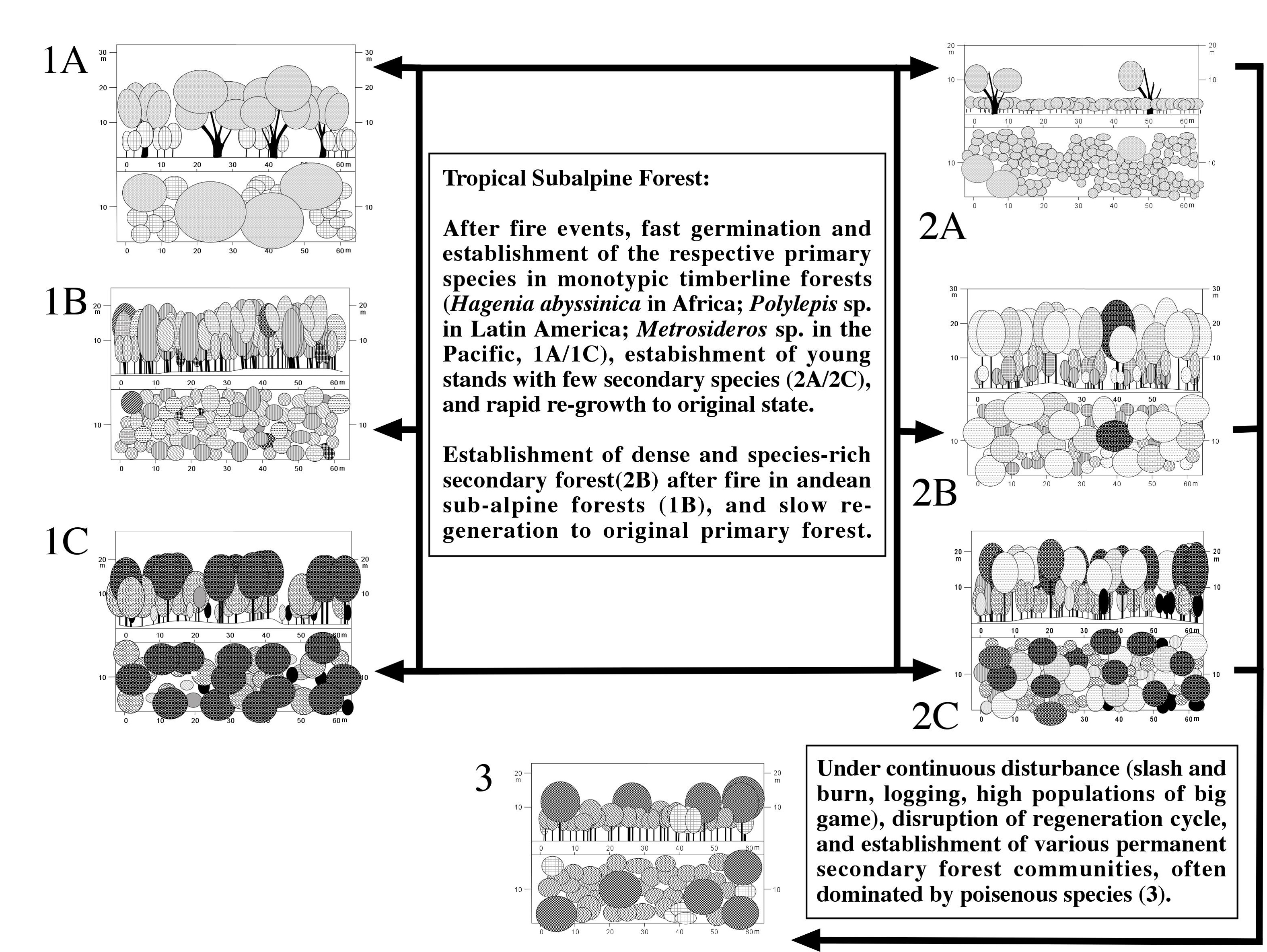
Figure 3:
Regeneration Processes in Tropical Subalpine Forests.
Figura 3: Procesos de regeneración en el Bosque Tropical Subalpino
Figura 3: Procesos de regeneración en el Bosque Tropical Subalpino
Tree heather
forests
On most of the east African mountains, the uppermost forests are constituted by tree heathers rather than by Hagenia forest. They show a surprising similarity among mountain ranges and would form the treeline at 3600-3900 m, if disturbance levels were lower (Wesche 2002b). Erica species burn exceedingly intense due to their small leaves, and fires often reach the rather low crowns. Most adult individuals survive, but vegetative regeneration takes years (Wesche 2002a). Given that the most widespread tree heathers Erica excelsa (southern Ethiopia E. trimera sl.) are capable to survive fire, whereas many other afromontane tree species are not, heather forests benefit from a moderate fire frequency (Hemp & Beck 2001). Thus they often form secondary replacement communities in the montane belt.
However, no other African tree species climbs as high as Erica excelsa and its relatives, so stands at the timberline cannot be regarded as replacement communities. This is nicely exemplified in the Rwenzori, where the most extensive heather forests are found, and where fires are certainly rare due to the almost permanently wet conditions.
In semi-humid mountain ranges, afroalpine vegetation dries out quickly in drought conditions, while the upper montane Hagenia forests retain some moisture in all but the most extreme years. Fires most commonly originate in the open vegetation, and not surprisingly, afroalpine vegetation and the bordering heather forests are much more frequently affected than Kosso forests. If fire intervals become short, time is not sufficient for regenerative recovery of adult Erica trees, nor can saplings grow big enough to develop a fire resistant bark. Thus, in the long run, Erica excelsa forests are replaced by more shrubby vegetation types or even afroalpine grasslands (Wesche et al. 2000). At present, timberlines have been depressed by 500 to 800 m due to burning.
Several succession schemes have been proposed (Schmitt 1991; Miehe & Miehe 1994b; Hemp & Beck 2001; Wesche 2002a), which all nicely demonstrate how Erica forests degenerate under frequent fires. However, a major shortcoming is the lack of information about the seedling stage, and about regeneration in the absence of disturbance. Except for the mostly shrubby E. arborea, no information on seed ecology is available, and sound data on re-sprouting are only available for Mt. Elgon (Wesche 2002a). At present, it is largely unclear, if E. excelsa, E. trimera and its relatives should be classified as re-seeders like the South African heathers or as re-sprouters like most of the Mediterranean species (Ojeda 1998; Pausas 2001). Thus, the available data are not sufficient to infer complete succession cycles, as they are available for other montane vegetation types.
Forest regeneration on tropical landslides
Naturally
occurring, often earthquake-triggered landslides are a crucial factor
for the dynamics and stability of tropical montane forest systems,
and are crucial for the maintenance of high species diversity. In
over-aged forest communities, particularly so in the Tropical Upper
Montane Forest, species diversity declines drastically (Ohl &
Bussmann 2004).
In East Africa, most montane forests are growing on relatively gentle slopes, and landslides are restricted to the steep flanks of river valleys, and are comparatively rare. After a slide event, the bare ground is rapidly colonized by the same pioneer species as in the Mosaic Climax regeneration cycle already described above.
In contrast, extremely steep slopes are the normal feature in the Andean and Pacific regions, and many other tropical mountains. Here, natural landslides are very common, and the main motor of forest regeneration. Lower Montane as well as Upper Montane Forests regenerate in a landslide induced succession cycle [[(Figure 4)]].
Landslides are extremely frequent in the tropical mountain regions. Destruction of roads and catastrophic events burying houses or even villages are common. Such slides, however, are usually initiated by human impact; most often by construction projects weakening the underground and by deforestation accelerating erosion. At some distance from roadsides and settlements, dense forests still exist. Even in these untouched areas, landslides are a very common phenomenon. Such natural slides are usually of smaller size than the anthropogenic slides. Investigations on landslide regeneration are scarce. Stern (1995) carried out research on the regeneration of a single landslide in Northern Ecuador (1995). Kessler (1999) studied succession on landslides in Bolivia, and Erickson et al. (1989) in the central and southern Andes. In other tropical mountain areas species colonization on landslides was analyzed by Garwood (1981 in Panama), Garwood et al. (1979), and Guariguata (1990 in Puerto Rico) and geomorphological processes by Batarya & Valdivia (1989 in the Lesser Himalaya in India), Restrepo & Vitousek (2001, Hawaii), Ohl & Bussmann (2004). Keefer (1984) studied earthquake triggered landslides all over the world.
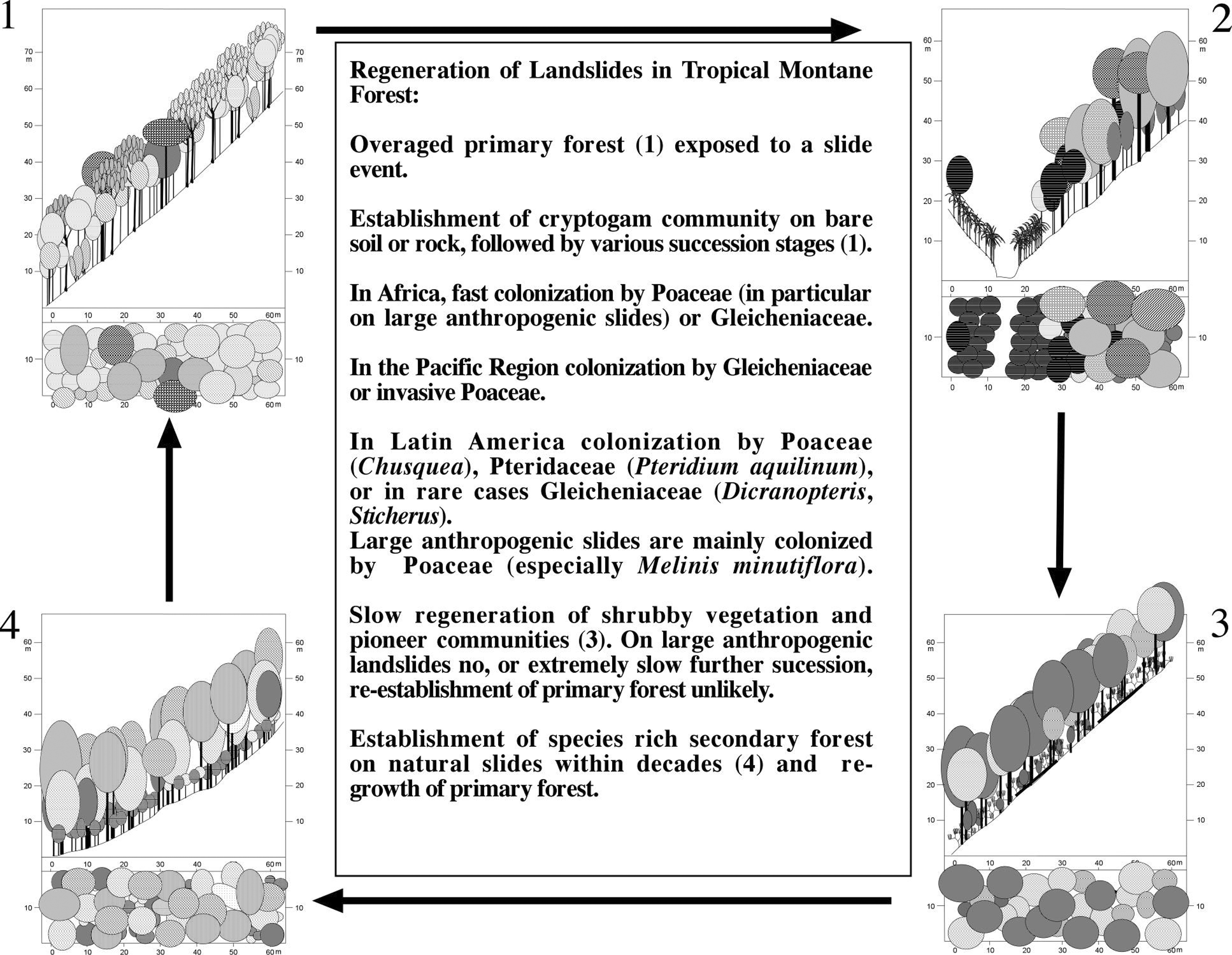
Figure 4:
Regeneration of tropical forest landslides.
Figure 4: Regeneración de derumbos en bosques tropicales.
Figure 4: Regeneración de derumbos en bosques tropicales.
The first remarkable thing noted when climbing on landslides, is the 'patchy' distribution of vegetation. The slides are very similar in shape, being long and narrow, although they vary in size. The surface is smooth and very few rocks are present. Inclination varies between about 30° and 80°. This leads to different erosive forces at different parts of the slide. Nevertheless, a direct correlation between vegetation cover and inclination or erosive energy would only partly account for the distribution of the vegetation. The study of soil cores of the slides under more, and less, dense vegetation did not produce results with significant differences in regard to soil texture, structure, color and pH. This excludes the edaphic conditions as principal responsible factors. Landslide areas are colonized quickly either at the borders of the slide or around islands that slipped down without being overturned due to vegetative propagation from the undisturbed neighboring areas and possibly due to a favorable microclimate. Other patches of high vegetation cover are created by the clonal, looping runner-shoot building growth of most of the individual pioneers that managed to establish seedlings first (Gleicheniaceae, Lycopodiaceae, Bambusoideae and Ericaceae). The majority of abundant species is wind-dispersed and produces many seeds. Under certain conditions freshly slipped slides do not last very long in the first stage and lichens and mosses do not develop well as the colonization by higher plants starts already in the first year of succession. On landslides well protected against wind and direct sunlight, seedlings of the surrounding flora established themselves after a few months. In contrast, a landslide exposed to wind and direct sunlight was bare of any vegetation about eight months after the slide event. Differences in vegetation along the altitudinal gradient have been found. This altitude corresponds to the change in the vegetation zonation in the surrounding forests (Bussmann 2001c). On the landslides at higher altitudes some species typical for alpine vegetation are found. Other distribution patterns do not correspond to vegetation changes along the altitudinal gradient but show similar patterns to differences in soil chemistry. Landslides are a common phenomenon in most tropical mountain systems. Stern (1995) and Kessler (1999) hypothesized that landslides maintain species diversity. Stern (1995) compares the effect of landslides to the meandering rivers of the lowland ecosystems. They create secondary forests dominated by colonizing species which are not able to survive in mature stands. Landslides are very common in some tropical areas where long and heavy rainfall and very steep slopes favor slides as well as a high number of more or less intense earthquakes. In addition, the building of roads and logging and burning weaken the substrate. The material and degree of weathering of the geological substrate is also important (Brabb & Harrold, 1989).
Species richness during the first two stages of regeneration is low due to the dominance of a few species of ferns or grasses. However, during the third stage of succession, species composition still differs somewhat completely to the surrounding forest, but diversity is high. The second stage with a dense cover of Gleicheniaceae has not been described from northern Ecuador (Stern 1995) but it was found on landslides in Bolivia (Kessler 1999) and Hawaii (Restrepo & Vitousek 2001). There, the role of Gleicheniaceae seems similar, although in Hawaii, the regeneration patterns are more and more influenced by invasive exotic species. Diplopterygium bancroftii and species of Sticherus dominate. In contrast, Stern (1995) found a dominant species of Chusquea, 31/2 years after the slide event at the lower zone of a landslide. She adds that the presence of the bamboo is especially noteworthy because under certain environmental conditions it can grow quickly and aggressively. Further on, she describes the great density of the bamboo, thereby having a profoundly limiting effect on the establishment of other plant species. The bamboo occurred at sites with a reasonable upper layer of organic debris. Other interesting differences in her work are that species of the genus Equisetum are important in the early stage and Blechnum dominates locally. No species of Gleicheniaceae were found. Reasons for those variations might be found in the obvious differences in geological substrate (of quaternary volcanic origin) and the lower humidity and altitude of the site (1440 m). Gleicheniaceae do not hinder the establishment of bushes, though the time period from when seedlings of bushes appear to when they manage to break through the fern layer, varies. Different types of succession models seem to correspond to the regeneration processes at the slides studies by Stern on one hand and on the other hand the slides observed by Kessler and the work on hand. Following the division of succession models according to Connell & Slayter (1977) and Pickett et al. (1987) the model of inhibition will have to be used to describe the situation in northern Ecuador as observed by Stern (1995). In contrast, the tolerance model combined with the facilitation model could be used to describe the situation in Bolivia (Kessler) and southern Ecuador. Little change in species composition but mainly a change in vegetation density or -height was observed due to local erosive energy, time elapsed since the last destruction, depth of the organic layer or the distance towards densely covered sites. Mosses and lichens are not only abundant during the first stage but also during the second and third stage and Gleicheniaceae are present in the second and third stage though they loose importance as they are overgrown by the bushes and trees of the pioneer forests. Up to this point, the model of tolerance seems to fit while the missing of species of the primary forests during the third stage follows the facilitation model. Under mature forest organic layers build up, but due to evaporation and transpiration they will not get heavily waterlogged. In contrast, comparable amounts of water do not transpire from senescent forest. A mosaic-like forest structure with younger and older forest stages is described in Kessler (1999) from the montane forest in the Bolivian Andes. He observed irregularly formed and spaced patches of senescent forest with single trees having already collapsed. This could explain the clustered occurrence of landslides as the risk of slipping in zones of senescent forest is higher than in zones of mature forest. In this case the effect of landslides in the ecosystem would be very important for the natural regeneration of the system. At altitudes above 2100 m, especially under senescent forest, very dense layers of terrestrial Bromeliads are found. Germination of other species is very difficult under these circumstances. In contrast, a landslide provides light and a high availability of minerals for successful plant growth.
What is the main difference between the studied natural landslides and the human triggered slides? Hartig (2000) carried out studies on the regeneration of the latter type of slides in Southern Ecuador. Antropogenic slides are usually very extensive. The surface is not smooth but often rocky. A heavy organic layer slipping over the mineral soil mainly creates natural slides. If thick mats of organic material become waterlogged due to long-lasting heavy rains, the weight of the material reaches a critical point when the adhesive strength gives in to gravity and a slide-event is initiated. The threshold in this area is very low as the adhesive strength is low due to the slippery mineral soil and the lack of a well developed rootsystem in the B-horizon which could help to fix the upper layers (own obs.; Stern 1995). The human triggered slides are usually initiated due to the weakened geological underground and have more in common with rock-falls. Succession differs between the two types of slides. Grasses largely replace the Gleicheniaceae and build a very dense layer often limiting the establishment of bush species. Succession seems to follow the inhibition model (Connell & Slayter 1977; Pickett et al. 1987). Especially the number of orchids is tremendous which leads to a very high diversity on man-made slides (Gross 1998). In contrast, there are not many species of orchids found at the natural slides but in the few areas with rocky relief they become more abundant.
Conclusions 
The
main formations of Tropical Montane Forests in Africa, Latin America
and the pacific share a lot of common characteristics in their
zonation and regeneration. Lower Montane Forests characteristically
form a diverse mosaic climax, where tree-fall represents the main
natural disturbance. Natural landslides and infrequent fires are the
main motors driving diversity maintenance, at least in mid-elevation
and high-altitude forests. All these forest formations tend to shift
towards less diverse, and possibly less stable degenerated secondary
forests under heavy human impact. Further research, especially in
Southeast Asia is required, to fully understand the global picture in
Tropical Mountain Forest Regeneration.
Acknowledgements 

I gratefully acknowledge the continuing support by Deutsche Forschungsgemeinschaft (DFG), and Bundesministerium fuer Bildung und Forschung (BmBF). Special thanks are due to all my counterparts from Bolivia, Cameroon, Ecuador, Ethiopia, French Polynesia, Germany, Kenya, Peru, Tanzania, and the USA.
References 

Agnew, A.D.Q. 1985. Cyclic sequences in plant communities of the Aberdare mountains, Kenya. J. East Afr. Nat. Hist. Soc. Natl. Mus. 75: 1-12.
Balslev, H., R. Valencia, G. Paz y Miño, H. Christensen & I. Nielsen 1998. Species count of vascular plants in one hectare of humid lowland forest in Amazonian Ecuador. In: Dallmeier, F. & J.A. Comiseky (Eds.). Forest biodiversity in North, Central and South America, and the Caribbean. Pearl River, Paris.
Banana, A.Y. & M. Tweheyo 2001. The ecological changes of Echuya afromontane bamboo forest, Uganda. Afr. J. Ecol. 39: 366-373.
Barnes, R.F.W. 1990. Deforestation trends in tropical Africa. Afr. J. Ecol. 28: 161-173.
Bartarya, S.K. & K.S. Valdiya 1989. Landslides and erosion in the catchment of the Gaula River, Kumaun Lesser Himalaya, India. Mountain Res. Dev. 9(4): 405-419.
Barthlott, W., W. Lauer & A. Placke 1996. Global distribution of species diversity in vascular plants: towards a world map of phytodiversity. Erdkunde 50: 317-327.
Brabb, E.E & B.L. Harrold 1989. Landslides: Extent and Economic Significance. Brookfield, Rotterdam.
Braun-Blanquet, J. 1964. Pflanzensoziologie (3rd edition). Springer, Wien, New York.
Buechner, H.K. & H.C. Dawkins 1961. Vegetation changes induced by elephants and fire in Murchinson Falls National Park, Uganda. Ecology 42: 752-766.
Bussmann, R.W. & E. Beck 1995a. The forests of Mount Kenya (Kenya), a phytosociological synopsis. Phytocoenologia 25 (5): 467-560.
Bussmann, R.W. & E. Beck 1995b. Regeneration- and cyclic processes in the Ocotea-Forests (Ocotea usambarensis Engl.) of Mt. Kenya. Verh. Ges. Ökol. 24: 35-38.
Bussmann, R.W. & E. Beck 1995c. Regeneration- and succession processes in the Cedar-Forests (Juniperion procerae) of Mt. Kenya. Ecotropica 1: 79-84.
Bussmann, R.W. 1997. The forest vegetation of the Harenna escarpment (Bale Province, Ethiopia) syntaxonomy and phytogeographical affinities. Phytocoenologia 27(1): 1-23.
Bussmann, R.W. 1999. Growth rates of important East African montane forest trees, with particular reference to those of Mt. Kenya. J. E. Afr. Nat. Hist. 88: 69-78.
Bussmann, R.W. & S. Lange 2000. Germination of important East African mountain forest trees. J E. Afr. Nat. Hist. 89: 101-111.
Bussmann, R.W. 2001a. Juniperus forests in Africa - Regeneration Ecology, Destruction and Possibilities for Management. Proceedings of the International Symposium "Problems of Juniper Forests: looking for solutions, Methods, Techniques", Osh, Kyrgysztan, 55-64.
Bussmann, R.W. 2001b. Succession and regeneration patterns of East African mountain forests. Syst. Geogr. Pl. 71: 959-974.
Bussmann, R.W. 2001c. The montane forests of Reserva Biológica San Francisco. Die Erde 132: 9-25.
Bussmann, R.W. 2002. Islands in the desert - forest vegetation of Kenya's smaller mountains and highland areas (Nyiru, Ndoto, Kulal, Marsabit, Loroghi, Ndare, Mukogodo, Porror, Mathews, Gakoe, Imenti, Ngaia, Nyambeni, Loita, Nguruman, Nairobi). J. E. Afr. Nat. Hist. 91 (1/2): 27-79, appendices 1-7 at www.naturekenya.org/JournalEANH.htm.
Bussmann, R.W. 2003. The vegetation of Reserva Biológica San Francisco, Zamora-Chinchipe, Southern Ecuador - a phytosociological synthesis. Lyonia 3(2): 145-254.
Bytebier, B. & R.W. Bussmann 2000. The vegetation of Mount Nyiru (Samburu, Kenya): A checklist and syntaxonomical survey. J. E. Afr. Nat. Hist. 89: 45-71.
Churchill, S.P. H. Balslev, E. Forero & J.L. Luteyn (Eds.) 1995. Biodiversity and Conservation of Neotropical Montane Forests. New York Botanical Garden, Bronx.
Connell, J.L. & R.O. Slayter 1977. Mechanisms of succession in natural communities and their role in community stability and organization. Am. Nat. 111: 1119-1144.
Dale, I.R. & P.J. Greenway 1961 Kenya trees and shrubs. Buchanan, Nairobi.
Demel, T. & A. Granström 1995. Soil seed banks in dry Afromontane forests of Ethiopia. J. Veg. Sci. 6: 777-786.
Demel, T. & A. Granström 1997a. Gemination ecology of forest species from the highlands of Ethiopia. J. Trop. Ecol. 14: 793-803.
Demel, T. & A. Granström 1997b. Seed viability of Afromontane tree species in forest soils. J. Trop. Ecol. 13: 81-95.
Demel, T. 1998. Soil seed bank at an abandoned Afromontane arable site. Feddes Rep. 109: 161-174.
Drake, D. & D. Mueller-Dombois 1993. Population Development of Rain-Forest Trees on a Chronosequence of Hawaiian lava Flows. Ecology 74(4): 1012-1019.
Ericksen, G.E., C.F. Ramirez, J.F. Concha, M.G. Tisnado & B.F. Urquidi 1989. Landslide hazards in the central and southern Andes. In Brabb, Harrod (Eds.) Landslides: Extent and economic significance. Balkema, Rotterdam.
Eshetu, Y. & K. Leinonen 2002. Seed germination response of four afromontane tree species to red/far-red ratio and temperature. For. Ecol. Mgt. 168: 53-61.
FAO 1992. Aménagement et conservation des forêts denses en Amérique tropicale. FAO. Etude Forêts 101. FAO, Rome.
FAO 1993. Summary of the final report of forest resource assessment 1990 for the tropical world. FAO, Rome.
Finegan, B. 1996. Pattern and process in neotropical secondary rain forest: the first 100 years of succession. Trends in Ecol. Evol. 11: 119-124.
Friis, I. 1992. Forests & Forest Trees of Northeast Tropical Africa. London, HMSO. Kew Bulletin Additional Series XV.
Garwood, N.C. 1981. Earthquake-caused landslides in Panama: recovery of the vegetation. - Res. Rep. Natl. Geogr. Soc. 21: 181-184.
Garwood, N.C., D.P. Janos & N. Brokaw 1979. Earthquake-caused landslides: A mayor disturbance in tropical forests. Science 205: 997-999.
Getachew, T. , T. Demel & M. Fetene 2002. Regeneration of fourteen tree species in Harenna forest, southeastern Ethiopia. Flora 197: 461-474.
Grimshaw, J. 1999. The afromontane bamboo, Yushania alpina, on Kilimanjaro. J. East Afr. Nat. Hist. 88: 69-78.
Gross, A.1998. Terrestrische Orchideen einer Hangrutschung im Bergwald Süd-Ecuadors: Verteilung, Phytomasse, Phänologie und Blütenmerkmale. Univ. Ulm, Diploma Thesis, unpubl.
Guariguata, M.R. 1990. Landslide disturbance and forest regeneration in the Upper Luquillo Mountains of Puerto Rico. J. Ecol. 78: 814-832.
Hamilton, L.S., J.O. Juvik & F.N. Scatena (Eds.) 1994. Tropical Montane Cloud Forests. Ecological Studies 110. Springer, New York.
Hammen, T. van der, A. Perez Precario & E.P. Pinto (Eds.) 1983. Studies on tropical andean ecosystems Vol. 1. Cramer, Vaduz.
Hammen, T. van der & R.M. Ruiz (Eds.) 1984. Studies on tropical andean ecosystems Vol. 2. Cramer, Vaduz.
Hammen, T. van der, S. Diaz-Piedrahita & V.J. Alvarez (Eds.) 1989a. Studies on tropical andean ecosystems Vol. 3. Cramer, Vaduz.
Hammen, T. van der, D. Mueller-Dombois & M.A. Little 1989b. Manual of Methods for Mountain Transect Studies. IUBS, Paris.
Hammen, T. van der & A.G. Dos Santo (Eds.) 1995. Studies on tropical andean ecosystems Vol. 4. Cramer, Vaduz.
Hartig, K. 2000. Pflanzensoziologische Untersuchungen von anthropogen gestörten Flächen im tropischen Bergwald Südecuadors. Univ. Bayreuth, Diploma Thesis, unpubl.
Hedberg, O. 1951. Vegetation belts of the East African mountains. Svensk Bot. Tidskr. 45: 140-199.
Hemp, A. & E. Beck 2001. Erica excelsa as a fire-tolerating component of Mt. Kilimanjaro's forests. Phytocoenologia 31: 449-475.
Holloway, C.W. 1965. The impact of big game on the forest policy in the Mount Kenya and North-East Aberdare forest reserves. E .Afr. Agric. For. J. 30(4): 370-389 .
Houston, M.A. 1995. Biological Diversity - The coexistance of species on changing landscapes. Cambridge University Press, Cambridge.
Hutchinson, J. 1967. Key to the families of flowering plants of the world. Oxford.
Ibisch, P.L. 1996. Neotropische Epiphytendiversität - das Beispiel Bolivien. Martina Galunder Verlag, Wiehl.
Katende, A.B., A. Birnie & Tengnas 1995. Useful trees and shrubs for Uganda. Identification, Propagation, and Management for agricultural and pastoral communities. Regional Soil Conservation Unit. Kampala, Nairobi. 710 pp.
Kebrom, T. & B. Tesfaye 2000. The role of soil seed banks in the rehabilitation of degraded hillslopes in southern Wello, Ethiopia. Biotropica 32: 23-32.
Keefer, D.K. 1984. Landslides caused by earthquakes. Geol. Soc. America Bulletin 95: 406-421.
Kerfoot, O. 1961. Juniperus procera Endl. (The African Pencil Cedar) in Africa and Arabia - I. Taxonomic affinities and geographical distribution. E. Afr. Agr. For. J. 26: 170-177.
Kessler, M.1999. Plant species richness and endemism during natural landslide succession in a perhumid montane forest in the Bolivian Andes. Ecotropica 5(2): 123-136.
Kessler, M. 2002. The Polylepis problem - where do we stand? - Ecotropica 8: 97-110.
Kitayama, K., E.G.G. Schuur, D. Drake & D. Mueller-Dombois 1997. Fate of a wet montane forest during soil ageing in Hawaii. J. Ecol. 85: 669-679.
Kortland, A. 1984. Vegetation research and the "bulldozer" herbivores of tropical Africa. In: Chadwick, A.C. & S.L. Sutton (Eds.) Tropical Rain-Forest. - The Leeds Symposium. Philosophical Society, Leeds
LaBastille A, Poole DJ (1978) On the need for a system of cloud-forest parks in Middle America and the Caribbean. Environmental Conservation 5(3): 183-190.
Lambrechts, C., B. Woodley, A. Hemp, C. Hemp & P. Nyiti 2002. Aerial Survey of the threats to Mt. Kilimanjaro forests, UNF, UNEP, KWS, Univ. Bayreuth, WCFT: 48.
Landon J.R. (Ed.) 1991. Booker tropical soil manual. - Essex, New York.
Lange, S.H., R.W. Bussmann & E. Beck 1997. Ecology and regeneration of the subalpine Hagenia abyssinica forests of Mt. Kenya. Bot. Acta 110(6): 473-480.
Legesse, N. 1993. Investigations on the germination behavior of wild olive seeds and the nursery establishment of the germinants. Sinet 16: 71-81.
Leigh, E.G. 1999. Tropical forest ecology - a view from Barro Colordo Island. Oxford University Press, Oxford.
Martin, R.E. 1982. Fire history and its role in succession. In: Means, J.E. (Ed.). Forest Succession and Stand Developement in the Northwest. Corvalis For. Res. Lab., Oregon State University.
Miehe, G. & S. Miehe 1994a. Zur oberen Waldgrenze in tropischen Gebirgen. Phytocoenologia 24: 43-110.
Miehe, S. & G. Miehe 1994b. Ericaceous forests and heathlands in the Bale Mountains of South Ethiopia - Ecology and man's impact. Stiftung Walderhaltung in Afrika. Hamburg. 206 pp.
Mueller-Dombois, D. & H. Ellenberg 200. Aims and methods of vegetation ecology. Wiley, New York.
Myers, N., R.A. Mittermeier, C.G. Mittermaier, G.A.B. da Fonseca & J. Kent 2000. Biodiversity hotspots for conservation priorities. Nature 403(25): 853-858.
Nieuwolt, S. 1978. Rainfall variability and drought frequencies in East Africa. Erdkunde 32: 81-88.
Ohl, C. & R.W. Bussmann 2004. Recolonisation of natural landslides in tropical mountain forests of Southern Ecuador. Feddes Rep. 115(3-4): 248-264.
Ojeda, F. 1998. Biogeography of seeder and re-sprouter Erica species in the Cape Floristic Region - Where are the resprouters? - Bio.l J. Linn. Soc. 63: 331-347.
Pausas, J.G. 2001. Resprouting vs. reseeding - a Mediterranean perspective. Oikos 94: 193-194.
Pickett, S.T.A. 1989. Space-for-time substitution as an alternative to long-term studies. In Likens, G.E. (Ed.). Long-term studies in ecology. New York, 110-135.
Pickett, S.T.A., S.L. Collins & J.J. Armesto 1987. Models, mechanisms and pathways of succession. Bot. Rev. 53: 335-371.
Plumptre, A.J. 1993. The effects of trampling damage by herbivores on the vegetation of the Parc National des Volcans, Rwanda. Afr. J. Ecol. 32: 115-121.
Reed, M. 1997. Effects of grazing and cultivation on afromontane plant communities on Mount Elgon, Uganda. Honors Thesis. University of Aberdeen. Aberdeen, 80.
Restrepo, C & P. Vitousek 2001. Landslides, Alien Species, and the Diversity of a Hawaiian Montane Mesic Ecosystem. Ecotropica 33(3): 409-420.
Schmitt, K. 1991. The vegetation of the Aberdare National Park, Kenya. Hochgebirgsforschung 7: 1-250.
Schmitt, K. & E. Beck 1992. Die Zerstörung eines Bergwalds in Kenia: Beispiele des Zusammenwirkens von Mensch und Großwild. Jahrb. Verein z. Schutze der Bergwelt 57: 207-226.
Scott, P. 1994. Bamboo in Mount Elgon, MECDP.
Sierra, R. (Ed.) 1999. Propuesta preliminar de un sistema de clasificación de vegetación para el Ecuador Continental. INEFAN/GEF, Quito.
Speck, H. 1986. Soils of the Mount Kenya Area, their formation, ecological, andagricultural significance. African Studies Series A1: 47-72.
Stadtmüller, T. 1987. Cloud forests in the humid tropics. A bibliographic review. United Nations University, Tokyo and CATIE, Turrialba.
Stern, M.J. 1995. Vegetation recovery on earthquake-triggered landslide sites in the ecuadorian Andes. In: Churchill S.P., H. Balslev, E. Forero & J.L. Luteyn (Eds.) Biodiversity and conservation of neotropical montane forests. Wiley, New York.
Stoyan, R. 2000. Aktivität, Ursachen und Klassifikation der Rutschungen in San Francisco/Südecuador. Univ. Erlangen, Diploma Thesis, unpubl.
Tamrat, B. 1993. Vegetation ecology of remnant Afromontane forests on the Central Plateau of Shewa, Ethiopia. Opulus Press AB. Uppsala.
Troll, C. 1959. Die tropischen Gebirge. Ihre dreidimensionale klimatische und pflanzengeographische Zonierung. Bonner Geogr. Abh. 25.
Wesche, K., G. Miehe & M. Kaeppelli 2000. The significance of fire for afroalpine ericaceous vegetation. Mountain Res. Dev. 20: 340-347.
Wesche, K. 2002a. The high-altitude environment of Mt. Elgon (Uganda/Kenya) - Climate, vegetation and the impact of fire. Ecotropical Monogr. 2: 1-253.
Wesche, K. 2002b. Structure and dynamics of Erica forest at tropical-African treelines. Verh. Reinh. Tüxen Ges. 14: 145-159.
Wesche, K. in press. The importance of occasional droughts for afroalpine landscape ecology. J. Trop. Ecol. 19.
White, F. 1978. The Afromontane Region. In: Werger, M.J.A. (Ed.) Biogeography and Ecology of Southern Africa. The Hague, Junk, pp 463-513.
White, F. 1983. The vegetation of Africa. Paris, Unesco.
Willan, R.L. 1965. Natural regeneration of high forest in Tanganyika. E. Afr. Agr. For. J. 31: 43-53.
Wimbush, S.H. 1937. Natural succession in the Pencil Cedar forests of Kenya Colony. Emp. For. J. 16: 49-53.
Wimbush, S.H. 1945. The african alpine Bamboo. Em.p For. J. 24: 33-39.
Winiger, M. 1979. Bodentemperaturen und Niederschlag als Indikatoren einer klimatisch-ökologischen Gliederung tropischer Gebirgsräume. Geomethodica 4: 121-15.
31