Introduction

For many years,
vegetation recovery was studied as an important topic in plant
ecology (Peet & Christensen 1980). The study of environmental
disturbances has a long research tradition, focusing on different
impacts, restoration and succession (White & Jentsch 2001). Some
studies suggest that natural disturbance plays an important role in
maintaining biodiversity (Christensen et al. 1989). These
processes of regeneration and succession are highly important in view
of increasing forest changes caused by natural or human activities.
Habitat fragmentation may increase the disturbance rates (Bergeron &
Brisson 1990).
Natural and
anthropogenic landslides are common all over the Andes including
Ecuador, and are producing serious and continuous damage (Benitez
1989). Unfortunately very few studies have been carried out in
Ecuador (Ohl & Bussmann 2004; Stern 1992; Benitez 1989).
Landslides are influenced by a series of internal (cryoclastic or
thermoplastic phenomena) and external environmental factors,
especially climate, slope, soil type, earthquake frequency and road
construction. These factors, sometimes combined, are the main driving
forces to produce debris and slides. The Andean mountains have always
been influenced by drastic climate processes, which directly
influence land movements and plant composition, as a part of a
natural dynamics. The disturbance regimens, have critical
implications in ecosystem maintenance, and influence species loss.
Native or alien species invasions play an important role in the
recovery process after landslides.
Discussion

Fall and Movement
In many mountain
ranges, continuing movements of material occur on steep slopes. "Rock
fall" is often used as the general term without further
reference to the material involved (Dikau et al. 1996). A fall occurs
when the natural slope exceeds the limit for the balance of the
material composing it. The fall may have various direct and indirect
natural or anthropogenic causes.
It is noteworthy to
consider that the Andes are characterized by active plate convergence
and uplift due to the South American Plate colliding with the Nazca
Plate (Van der Hammen 1988). Intense seismic activity occurs
constantly. The uplift average is as much as a meter per thousand
years, and rapid erosion has resulted in widespread unstable slopes
(Eriksen et al. 1989). The way landslides move and their
velocity have inter-relations with the nature of the fall, size and
provenance of the material. Different fall classifications based on
either genetic or descriptive approaches are used (Whalley 1974). The
most common international classification is based on the origin and
nature of the material, although many other descriptions and
suggestions exist. Terzaghi (1962) for example describes falls
according to the rock type, focusing on the rupture mechanism and the
action of water in the rock.
Slide and Landslide
The term landslide
is used for a movement of material along a recognizable shear surface
(Buma & Van Asch 1996). At least two different kinds of slides
are recognized: Rotational, which basically describes how, after
failure is initiated, the slump mass starts to rotate; and
translational, which is not a circular failure, but a movement
largely controlled by surface of weakness within the structure of the
slope-forming material. Translational slides may occur in three types
of material: rock, debris and soil. Depending on the slope angle and
the velocity, slides will either stay as a discrete block on the
failure surface or break into debris. According to Varnes (1978),
cited in Dikau et al. (1997), show the following classification
(Table 1):
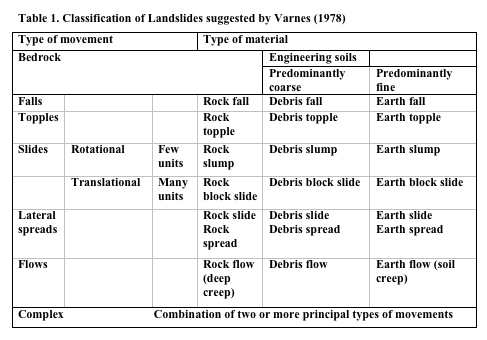
Table 1. Classification of Landslides suggested by Varnes (1978).
Disturbances and Species Loss
Different kinds of
disturbances, such as fire, landslides, flooding, grazing among
others change the biological organization of ecosystems.
"Disturbances play a crucial role in maintaining biotic
diversity" (Darwin 1859). Species evolve a diverse spectrum of
abilities relative to disturbance (Vogl 1974). After a particular
disturbance, some species increase in number or invade, while others
decrease or retreat (Walker et al. 1999). Dominant and minor
species can occur in the same functional group and can be similar
with respect to their contribution to ecosystem function. Dominant
and less dominant species switch in abundance under changing
environmental conditions allowing "functional stability".
Latin America has
the largest extend of all tropical forest (Whitmore 1997), but also
the highest deforestation rate, with approximately 32 million ha or
0.96%/yr. Plant diversity in the Ecuadorian Andes is considered
extreme with a high degree of endemism (Lozano et al. 2003;
Lozano & Bussmann in prep), probably due to the composition of
different lineages, and also influenced by environmental as well as
geographic factors (Richter 2003). Many mountain plant species are
restricted to narrow and specific elevation ranges (Young 1994),
allowing explosive radiation as reported by Gentry (1982) and Jost
(2004). The best examples are the narrow altitudinal belts of the
"San Francisco Reserve" in the elfin forest, where
Bussmann (2002) reports an extremely diverse community complex.
Disturbance in areas with such speciation conditions affects a whole
"micro-ecosystem" and requires "complex"
processes to recover again.
During the last
decades, tropical American forests are rapidly altered and
disappearing, with logging statistics showing a loss of 2.6 millions
ha/year. Their extreme biodiversity richness is changing and high
species loss occurs, with long term effects difficult to predict.
No data exist on the
exact deforestation rate in Ecuador. Data suggest a forest loss to
deforestation of 136.000 to 340.000 ha/year (FAO 2001). South Ecuador
does not show a very high rate of deforestation because most of the
logging is concentrated in the North and West of the country. A high
amount of logging occurs however in the South as well.
Succession
Succession of
vegetation usually follows natural and anthropogenic processes. The
term succession is used to describe many types of vegetation changes
on widely different scales in both space and time (Finegan 1984).
Earliest studies describe the sequence of species that successively
invade a site (Cooper 1913), show changes in Biomass, productivity,
diversity and niche (Odum 1969), while others have focused on
physical stresses to plants and competition for resources between
plants as the main mechanisms determining the course of succession
(Colinvaux 1973). Therefore competitive interactions with herbivores,
predators, and pathogens are of critical importance to the course of
succession (Connell & Slatyer 1977). The high primary net
productivity of successional ecosystem can support large animal
populations (Linares 1976), hence such standing patch interaction
serve as important nuclei of species establishment during succession
process.
Factors of the
physical environment (light, temperature, soil, relative humidity)
triggerecophysiological responses, for example seeds if pioneer
species in soil seed banks take advantage of disturbance enhancing
the optimal conditions of early successional plants. Few data and
comparative studies of fragmented ranges exit (Young 1994).
Distribution and size of patches probably reflect seed dispersal more
than site differences (Ewel 1983). Other considerations on succession
are described by Richter (2003), specifically for Southern Ecuador.
There, climatic conditions, mainly humidity and physiography of
mountain chains, depict micro-site and environments varieties. These,
combined with regimens of frequent and different sized disturbance,
especially "landslides", can be considered optimum spots
for a high rate of genetic exchange as consequence of
micro-geographic niche partitioning.
Succession
is important for two reasons: the value of the concept in the
development of ecology as a science and it's enormous potential
in the development of programs for the conservation and exploitation
of biological resources (Richards, 1976 cited in Finegan, 1984).
The pioneer
vegetation on landslides in South Ecuador shows often a high number
of species. Fifty six families, 127 genera and 264 species were found
on natural landslides, while man-made landslides had 69 plant
families, 127 genera and 313 species (Lozano & Bussmann 2005).
Anthropogenic Disturbance and Economical
Significance
From an engineer
point of view, mountains offer very difficult conditions for road
construction and maintenance (Young 1994). Because road maintenance
is politically unattractive compared to the establishment of new
roads, minimal efforts are made to maintain existing infrastructure.
The socio-economical expenses after "landslides"
triggered by road construction have been studied in St. Vincent, St.
Lucia and Dominica, where the average annual cost for landslide
damage to roads range from $115,000 to $121,000 in normal years (De
Graff et al. 1989). The average annual cost of landslide
investigation, repair and maintenance in the larger islands of
Trinidad and Tobago are $1,26 millions and $0,96 millions,
respectively. In an average year the cost of repairing landslide
damage to roads throughout the Caribbean amounts to $15 million.
Ericksen et al. (1989), indicate that in the central and southern
Andes the average annual property damage is on the order of few
millions to several tens of millions of dollars. Major landslides,
which occur at 5- to 10-year intervals, may however cause property
damage of hundreds of millions of dollars, in addition to personal
insurance claims involved.
Stern
(1992) describes how earthquakes caused a nationwide socio-economic
tragedy and environmental disaster on Mach 5, 1987 in Northeastern
Ecuador. Thousands of human lives and countless homes were lost in
the aftermath of landslide and floods, Chávez and Lara (1989)
estimate 400000000 m3 of debris material and Figueroa et
al. (1987) reported up to 6000000000 m3 of
landslide-related silt and debris was deposited in and transported
downstream by the Aguarico and Coca rivers. Thirty-three km of the
trans-Ecuadorian oil pipeline and 45 km of the natural gas pipeline
were destroyed, and it took eight month repair the damage. Benitez
(1989) reports that a landslide near Chunchi 1983 blocked the Pan
American Highway, seriously damaged homes, and a loss of 150 lives
was the final result of this tragedy. The social economic impact
related to cattle-raising and agriculture activities, without
considering life insurance claims, exceed $4 millions on the past
decade. In southern Ecuador "landslides" continuously
damage roads, especially on the way to Zamora and Valladolid. This
has led to serious disasters and large economical expenses.
Conclusions

Landslides
at the Ecuadorian Andes are wide-spread, because of unstable slopes
combined with environmental conditions as well as inappropriate road
construction and other anthropogenic factors. Millions of dollars
have been expended during the lasts decades in the mitigation of
landslides hazards in Ecuador alone. The disturbance of watersheds
still continues, without any mitigation plan or conservation
measures, and no strategies for forest preservation and restoration
are included in the national policy.
In
cloud forests succession of gaps starts with a slow cover of mosses
and other cryptogams, followed by herbs, shrubs and trees arriving in
the final stages. Many mountain plant species are restricted to
narrow and specific elevation ranges. The species richness in
southern Ecuador indicates high amount of pioneer plants in
regeneration. It is however still not sufficiently understood if
perturbations act as a motor for the maintenance of biodiversity.
Fragmentation
by natural and anthropogenic disturbance regimens, especially
landslides in the Ecuadorian Andes, seems usually to be linked to
legal and economical factors. Policy evaluations need to include
criteria such as social requirements, and tools of environmental
management must be applied to a constant landslide mitigation plan
effort maintain biodiversity.
Acknowledgements

We
thank the German Science Foundation (DFG) for support of this
project (DFG FOR 402-1/1 TP7 and FOR 402-2/1 A2, as well as the
National University of Loja, Department of Botany and Ecology,
Herbarium LOJA.
References

Benitez, A. 1989.
Extend and Economic Significance in Ecuador. Brabb,
E.E. & B.L. Harrod (Eds.). Landslides: Extent and Ecologycal
Significance. Balkema, Rotterdam.
Bergeron,
Y. & J. Brisson. 1990. Fire regime in red pine stands at
the northern limit of the species range. Ecology 71, 1352-1364.
Buma,
J. & T. Van Asch. 1996. Slide Rotational 4: 43-61.
In: Dikau, R.; D. Brunsden; L. Schrott & M.
Ibsen (Eds.). Landslide Recognition. Report No. 1 of
the European Commission Environment Programme Contract No.
EV5V-CT94-0454. Identification, Movement and Causes. Chichester.
Pp. 251.
Bussmann,
R.W. 2002. Estudio Fitosociológico de la Vegetación
en la Reserva Biológica de San Francisco (ECSF), Zamora
Chinchipe. Herbario Loja 8.
Chávez,
M.A. & O. Lara. 1989. Análisis de los deslizamientos
catastróficos Producidos por los Sismos de marzo 5 de 1987.
1° Simposio Suramericano de Deslizamientos, 7-10 agosto, Paipa,
Colombia.
Christensen,
N.L.; J.K. Agee; P.F Brussard,.; J. Hughes; D.H. Knight; G.W.
Minshall; J.M. Peek; S.J. Pyne; F.J. Swanson; J.W. Thomas; S. Wells;
S.W. Williams & H.A. Wright. 1989. Interpreting the
Yellowstone fires of 1988. Bioscience 39, 678-685.
Colinvaux, P.A.
1973. Introduction to Ecology. Wiley, New York. 621 pp.
Connell, J.H. &
R.O. Slatyer. 1977. Mechanism of Succession in Natural Communities
and Their Role in Community Stability and Organization. Amer.
Natur. 111, 1119-1114.
Cooper, W. S. 1913.
The climax forest of Isle Royale, Lake superior, and it's
development. Bot. Gaz. 55: 1-235.
Darwin, C. 1859.
On the Origin of Species by means of natural selection. John
Murray, London.
De
Graff, V.J.; R. Bryce; R.W. Jibson; S. Mora & C.T. Rogers.
1989. Landslides: Their Extend and Significance in the Caribbean. In:
Brabb E.E. & B.L. Harrold (Eds.) Landslides:
Extent and Ecologycal Significance. Balkema,
Rotterdam
Dikau,
R.; D. Brunsden; L. Schrott & M. Ibsen (Eds.) 1996. Landslide
Recognition. Report No. 1 of the European Commission Environment
Programme Contract No. EV5V-CT94-0454. Identification, Movement and
Causes. Chichester. Pp. 251.
Erikson, G.E.; C.F.
Ramirez; J.F. Concha; M.G. Tisnado & B.F. Urquidi. 1989.
Landslides hazard in the central and southern Andes: 111-117. In:
Brabb, E.E. & B.L. Harrold (Eds.). Landslides: Extent and
Ecologycal Significance. Balkema, Rotterdam.
Ewel,
J. 1983. Succession. In: Ecosystem of the World 14A Tropical Rain
Forest Ecosystems. Structure and Functions. Golley, F.B.
(Ed.). Elsevier Scientific Publishing Company. New York. 13, 217-223.
FAO.
2001. Estado de la Información Forestal en el Ecuador,
Información para el Desarrollo Forestal Sostenible
Monografía de Países, Volumen 7, Santiago, Chile.
Figueroa,
E.; G. Oviedo; C. Vela; R. Sierra; H. Balslev; J. Torres; A. Carrasco
& T. de Vries. 1987. Evaluación del Impacto Ambiental
del sismo en la Amazonia. Fundación Natura. Quito,
Ecuador.
Finegan, B. 1984.
Forest Succession. Nature 312, 109-113.
Gentry,
A. 1982. Neotropical Floristic Diversity: Phytogeographycal
Connections Between Central and South America, Pleistocene Climatic
Fluctuations, or an Accident of the Andean Orogeny? Ann. Missouri
Botanical Garden 71, 273-295.
Jost, L. 2005.
Explosive Local Radiation of the Genus Teagueia (Orchidaceae)
in the Upper Pastaza Watershed of Ecuador. In:
Lozano, P.; R.W. Bussmann & H. Navarrete (Eds). Memorias del
II Congreso de Biodiversidad de los Andes y Amazona y IV Ecuatoriano
de Botánica. Fundación Ecuatoriana Para la
Investigación de La Botánica FUNBOTANICA, Loja-Ecuador.
Linares, O.F. 1976.
"Garden hunting" in the American tropics. Human Ecol.
4, 331-349
Lozano, P. &
R.W. Bussmann. 2005 (In press). Importancia de los
deslizamientos en el Parque Nacional Podocarpus, Loja-Ecuador.
Revista Peruana de Biología 12 (2).
Lozano,
P.; T. Delgado & Z. Aguirre M. 2003. Estado Actual de la Flora
Endémica Exclusiva y su Distribución en el Occidente
del Parque Nacional Podocarpus. Publicaciones de la Fundación
Ecuatoriana para la Investigación y Desarrollo de la Botánica.
Loja, Ecuador.
Odum, E.P. 1969. The
strategy of ecosystem development. Science. 164, 262-270.
Ohl, C. & R.W.
Bussmann. 2004. Recolonisation of Natural
Landslides in Tropical Mountains Forest of Southern Ecuador. Feddes
Repertorium 115 (3-4). 248-264.
Peet, R.K. & L.
Christensen. 1980. Succession: A Population Process. Vegetation
43, 131-140.
Richards, P.W. 1976.
The Tropical Rain Forest. Cambridge University Press.
Richter, M. 2003.
Using Epiphytes and Soil Temperatures for Eco-Climatic Interpretation
in Southern Ecuador. ERDKUNDE 57, 161-181.
Stern, M.J. 1992.
Ecosystem Response to Natural and Anthropogenic Disturbances in
the Andean Cloud Forest of Ecuador. Ph.D. Thesis. University of
California.
Terzaghi, K. 1962.
Stability of steep slops on hard unweathered rock. Geotechnique
12, 251-270.
Van
der Hammen, T. 1988. South America. Vegetation
History. Kluwer Academic Publisher
Vogl, R.J. 1974.
Effects of fire on grassland. In: Kozlowski T.T. & C.E. Ahlgren
(Eds.) Fire and Ecosystems. Academy Press, New York, pp.
139-194.
Walker, B.; A.
Kinzing & J. Langridge. 1999. Plant attribute diversity,
resilience, and ecosystem function: the nature and significance of
dominant and minor species. Ecosystems 2, 95-113.
Whalley, W.B. 1974.
The mechanics of high magnitude low frequency rock failure and its
importance in a mountainous area. Geogr. Papers, Reading
University. 27, p. 48.
White, P. & A.
Jentsch. 2001. The Search for Generality in Studies of Disturbance
and Ecosystem Dynamics. Progress in Botany
62, 399-449.
Whitemore,
T.C. 1997. Tropical Forest Disturbance, Disappearance, and Species
Loss Pp.3-12 In: Laurence, W. & R. Bierregaard (Eds.). Tropical
Forest Remnants. The University of Chicago Press, London.
Young,
K. 1994. Roads and Environmental Degradation of Tropical Montane
Forest. Conservation Biology 8 (4), 972-976.